Big galaxies at high redshift!
That’s my prediction, anyway. A little context first.
New Year, New Telescope
First, JWST finally launched. This has been a long-delayed NASA mission; the launch had been put off so many times it felt like a living example of Zeno’s paradox: ever closer but never quite there. A successful launch is always a relief – rockets do sometimes blow up on lift off – but there is still sweating to be done: it has one of the most complex deployments of any space mission. This is still a work in progress, but to start the new year, I thought it would be nice to look forward to what we hope to see.
JWST is a major space telescope optimized for observing in the near and mid-infrared. This enables observation of redshifted light from the earliest galaxies. This should enable us to see them as they would appear to our eyes had we been around at the time. And that time is long, long ago, in galaxies very far away: in principle, we should be able to see the first galaxies in their infancy, 13+ billion years ago. So what should we expect to see?
Early galaxies in LCDM
A theory is only as good as its prior. In LCDM, structure forms hierarchically: small objects emerge first, then merge into larger ones. It takes time to build up large galaxies like the Milky Way; the common estimate early on was that it would take at least a billion years to assemble an L* galaxy, and it could easily take longer. Ach, terminology: an L* galaxy is the characteristic luminosity of the Schechter function we commonly use to describe the number density of galaxies of various sizes. L* galaxies like the Milky Way are common, but the number of brighter galaxies falls precipitously. Bigger galaxies exist, but they are rare above this characteristic brightness, so L* is shorthand for a galaxy of typical brightness.
We expect galaxies to start small and slowly build up in size. This is a very basic prediction of LCDM. The hierarchical growth of dark matter halos is fundamental, and relatively easy to calculate. How this translates to the visible parts of galaxies is more fraught, depending on the details of baryonic infall, star formation, and the many kinds of feedback. [While I am a frequent critic of model feedback schemes implemented in hydrodynamic simulations on galactic scales, there is no doubt that feedback happens on the much smaller scales of individual stars and their nurseries. These are two very different things for which we confusingly use the same word since the former is the aspirational result of the latter.] That said, one only expects to assemble mass so fast, so the natural expectation is to see small galaxies first, with larger galaxies emerging slowly as their host dark matter halos merge together.
Here is an example of a model formation history that results in the brightest galaxy in a cluster (from De Lucia & Blaizot 2007). Little things merge to form bigger things (hence “hierarchical”). This happens a lot, and it isn’t really clear when you would say the main galaxy had formed. The final product (at lookback time zero, at redshift z=0) is a big galaxy composed of old stars – fairly typically for a giant elliptical. But the most massive progenitor is still rather small 8 billion years ago, over 4 billion years after the Big Bang. The final product doesn’t really emerge until the last major merger around 4 billion years ago. This is just one example in one model, and there are many different models, so your mileage will vary. But you get the idea: it takes a long time and a lot of mergers to assemble a big galaxy.
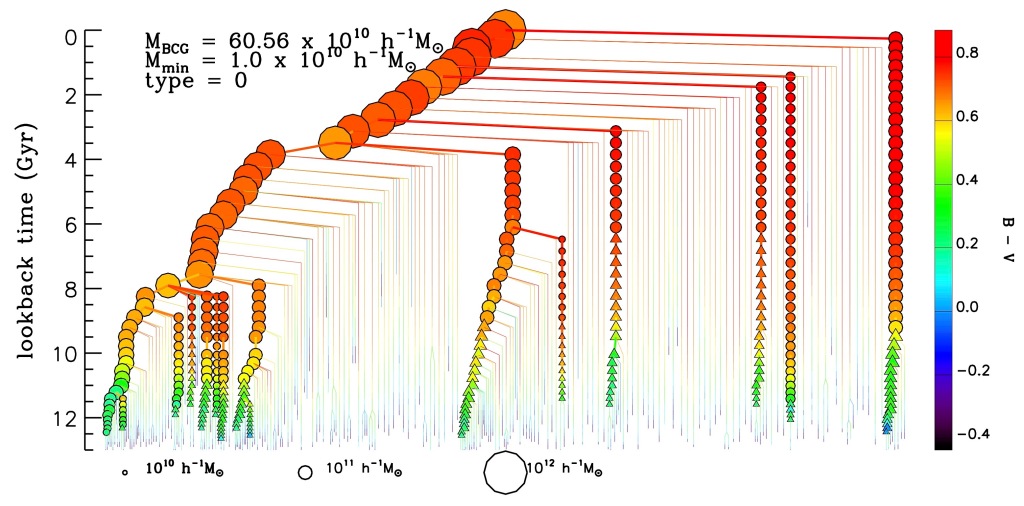
It is important to note that in a hierarchical model, the age of a galaxy is not the same as the age of the stars that make up the galaxy. According to De Lucia & Blaizot, the stars of the brightest cluster galaxies
“are formed very early (50 per cent at z~5, 80 per cent at z~3)”
but do so
“in many small galaxies”
– i.e., the little progenitor circles in the plot above. The brightest cluster galaxies in their model build up rather slowly, such that
“half their final mass is typically locked-up in a single galaxy after z~0.5.”
De Lucia & Blaizot (2007)
So all the star formation happens early in the little things, but the final big thing emerges later – a lot later, only reaching half its current size when the universe is about 8 Gyr old. (That’s roughly when the solar system formed: we are late-comers to this party.) Given this prediction, one can imagine that JWST should see lots of small galaxies at high redshift, their early star formation popping off like firecrackers, but it shouldn’t see any big galaxies early on – not really at z > 3 and certainly not at z > 5.
Big galaxies in the data at early times?
While JWST is eagerly awaited, people have not been idle about looking into this. There have been many deep surveys made with the Hubble Space Telescope, augmented by the infrared capable (and now sadly defunct) Spitzer Space Telescope. These have already spied a number of big galaxies at surprisingly high redshift. So surprising that Steinhardt et al. (2016) dubbed it “The Impossibly Early Galaxy Problem.” This is their key plot:

There are lots of caveats to this kind of work. Constructing the galaxy luminosity function is a challenging task at any redshift; getting it right at high redshift especially so. While what counts as “high” varies, I’d say everything on the above plot counts. Steinhardt et al. (2016) worry about these details at considerable length but don’t find any plausible way out.
Around the same time, one of our graduate students, Jay Franck, was looking into similar issues. One of the things he found was that not only were there big galaxies in place early on, but they were also in clusters (or at least protoclusters) early and often. That is to say, not only are the galaxies too big too soon, so are the clusters in which they reside.
Dr. Franck made his own comparison of data to models, using the Millennium simulation to devise an apples-to-apples comparison:

The result is that the data look more like big galaxies formed early already as big galaxies. The solid lines are “passive evolution” models in which all the stars form in a short period starting at z=10. This starting point is an arbitrary choice, but there is little cosmic time between z = 10 and 20 – just a few hundred million years, barely one spin around the Milky Way. This is a short time in stellar evolution, so is practically the same as starting right at the beginning of time. As Jay put it,
“High redshift cluster galaxies appear to be consistent with an old stellar population… they do not appear to be rapidly assembling stellar mass at these epochs.”
Franck 2017
We see old stars, but we don’t see the predicted assembly of galaxies via mergers, at least not at the expected time. Rather, it looks like some galaxies were already big very early on.
As someone who has worked mostly on well resolved, relatively nearby galaxies, all this makes me queasy. Jay, and many others, have worked desperately hard to squeeze knowledge from the faint smudges detected by first generation space telescopes. JWST should bring these into much better focus.
Early galaxies in MOND
To go back to the first line of this post, big galaxies at high redshift did not come as a surprise to me. It is what we expect in MOND.
Structure formation is generally considered a great success of LCDM. It is straightforward and robust to calculate on large scales in linear perturbation theory. Individual galaxies, on the other hand, are highly non-linear objects, making them hard to beasts to tame in a model. In MOND, it is the other way around – predicting the behavior of individual galaxies is straightforward – only the observed distribution of mass matters, not all the details of how it came to be that way – but what happens as structure forms in the early universe is highly non-linear.
The non-linearity of MOND makes it hard to work with computationally. It is also crucial to how structure forms. I provide here an outline of how I expect structure formation to proceed in MOND. This page is now old, even ancient in internet time, as the golden age for this work was 15 – 20 years ago, when all the essential predictions were made and I was naive enough to think cosmologists were amenable to reason. Since the horizon of scientific memory is shorter than that, I felt it necessary to review in 2015. That is now itself over the horizon, so with the launch of JWST, it seems appropriate to remind the community yet again that these predictions exist.
This 1998 paper by Bob Sanders is a foundational paper in this field (see also Sanders 2001 and the other references given on the structure formation page). He says, right in the abstract,
“Objects of galaxy mass are the first virialized objects to form (by z = 10), and larger structure develops rapidly.”
Sanders (1998)
This was a remarkable prediction to make in 1998. Galaxies, much less larger structures, were supposed to take much longer to form. It takes time to go from the small initial perturbations that we see in the CMB at z=1000 to large objects like galaxies. Indeed, the it takes at least a few hundred million years simply in free fall time to assemble a galaxy’s worth of mass, a hard limit. Here Sanders was saying that an L* galaxy might assemble as early as half a billion years after the Big Bang.
So how can this happen? Without dark matter to lend a helping hand, structure formation in the very early universe is inhibited by the radiation field. This inhibition is removed around z ~ 200; exactly when being very sensitive to the baryon density. At this point, the baryon perturbations suddenly find themselves deep in the MOND regime, and behave as if there is a huge amount of dark matter. Structure proceeds hierarchically, as it must, but on a highly compressed timescale. To distinguish it from LCDM hierarchical galaxy formation, let’s call it prompt structure formation. In prompt structure formation, we expect
- Early reionization (z ~ 20)
- Some L* galaxies by z ~ 10
- Early emergence of the cosmic web
- Massive clusters already at z > 2
- Large, empty voids
- Large peculiar velocities
- A very large homogeneity scale, maybe fractal over 100s of Mpc
There are already indications of all of these things, nearly all of which were predicted in advance of the relevant observations. I could elaborate, but that is beyond the scope of this post. People should read the references* if they’re keen.
*Reading the science papers is mandatory for the pros, who often seem fond of making straw man arguments about what they imagine MOND might do without bothering to check. I once referred some self-styled experts in structure formation to Sanders’s work. They promptly replied “That would mean structures of 1018 M☉!” when what he said was
“The largest objects being virialized now would be clusters of galaxies with masses in excess of 1014 M☉. Superclusters would only now be reaching maximum expansion.”
Sanders (1998)
The exact numbers are very sensitive to cosmological parameters, as Sanders discussed, but I have no idea where the “experts” got 1018, other than just making stuff up. More importantly, Sanders’s statement clearly presaged the observation of very massive clusters at surprisingly high redshift and the discovery of the Laniakea Supercluster.
These are just the early predictions of prompt structure formation, made in the same spirit that enabled me to predict the second peak of the microwave background and the absorption signal observed by EDGES at cosmic dawn. Since that time, at least two additional schools of thought as to how MOND might impact cosmology have emerged. One of them is the sterile neutrino MOND cosmology suggested by Angus and being actively pursued by the Bonn-Prague research group. Very recently, there is of course the new relativistic theory of Skordis & Złośnik which fits the cosmologists’ holy grail of the power spectrum in both the CMB at z = 1090 and galaxies at z = 0. There should be an active exchange and debate between these approaches, with perhaps new ones emerging.
Instead, we lack critical mass. Most of the community remains entirely obsessed with pursuing the vain chimera of invisible mass. I fear that this will eventually prove to be one of the greatest wastes of brainpower (some of it my own) in the history of science. I can only hope I’m wrong, as many brilliant people seem likely to waste their career running garbage in-garbage out computer simulations or at the bottom of a mine shaft failing to detect what isn’t there.
A beautiful mess
JWST can’t answer all of these questions, but it will help enormously with galaxy formation, which is bound to be messy. It’s not like L* galaxies are going to spring fully formed from the void like Athena from the forehead of Zeus. The early universe must be a chaotic place, with clumps of gas condensing to form the first stars that irradiate the surrounding intergalactic gas with UV photons before detonating as the first supernovae, and the clumps of stars merging to form giant elliptical galaxies while elsewhere gas manages to pool and settle into the large disks of spiral galaxies. When all this happens, how it happens, and how big galaxies get how fast are all to be determined – but now accessible to direct observation thanks to JWST.
It’s going to be a confusing, beautiful mess, in the best possible way – one that promises to test and challenge our predictions and preconceptions about structure formation in the early universe.
Fingers still crossed.
I would repeat my prediction of several months ago, that the entire premise of a temporally finite, expanding universe will go the way of super symmetry, as those distant galaxies resolve into mature galaxies, shifted off the visible spectrum and even more distant ones are detected, such that the background radiation appears as the solution to Olber’s paradox, the light of infinite sources.
Obviously this puts me in the crank category, so I won’t try anyone’s patience beyond that.
LikeLike
Thirty years ago HST sparked hopes that cosmology and cosmologists would change for the better. With observations of galaxies “too mature to exist in the early universe”, comsology changed but cosmologists remained stubbornly set on the model they want to protect at all costs: more dark matter, more dark energy, more black holes, more Bang, more parameters, more hocus pocus than can be found in the best ‘Handbook of Magic’.
At least MOND proponents are trying to get rid of some of the magic formulas. They deserve high praise for that.
What will JWST see? More massive galaxies with smaller radii, mature looking but with star forming rates that exceed any currently understood limits (assuming observations of high redshift objects are interpreted in an expanding universe paradigm). Current observations are already a source of several tensions in cosmology. JWST observations will also challenge both LCDM and MOND, but few astronomers will be willing to abandon these models.
LikeLiked by 1 person
I was hoping the same. Yet they managed to shoehorn everything in. It would do any cult proud.
Though I think it will crack this time. Not that the True Believers will give up, but something akin to String Theory, where it becomes obvious it’s flawed, even if the field is not yet ready to move on. The fact is much of the current age limits, as well as the large scale structures have to be either squeezed into the lower ranges, or ignored, so if observations definitively push these out, the cracks are going to get real. Explaining it in 13.8 billion years will be like trying to explain the earth in the 6000 years of the Bible.
I suspect there is a deeper issue as well, in that the only way I’ve heard that light will redshift over distance alone is as “packets,” as the higher frequencies dissipate faster. Therefore we are sampling a wave front, not detecting individual photons traveling billions of lightyears. So that would mean the quantization of light is a function of the absorption of it by the detecting and measuring devices, not fundamental to the light itself. The “loading” theory of light.
Imagine how that would go down, with the larger theoretical physics community.
An interesting opinion piece, from some years ago;
https://www.americanscientist.org/article/modern-cosmology-science-or-folktale
LikeLike
I found this recent Youtube, by Eric Reiter, presenting the history of the loading theory;
LikeLike
Jay Frank? typo? Franck?
LikeLiked by 1 person
D’oh! Thanks. I thought I caught all of those. Maybe autocorrect out corrected itself.
LikeLike
We can only hope that the JWST will serve the role of the Michelson-Morley experiment which removed the props under the concept of the aether, allowing in due time Einstein’s Theory of Special Relativity to be recognised as correct. I note that the famous experiment was performed at your very own institution, so perhaps this will be the second time it has played a role at a crucial point in physics.
LikeLike
One can hope. But this comes back to the eternal question: what could falsify dark matter? This could be the subject of a whole post, or book, but it seems that the psychology is to not consider MOND until dark matter is in serious doubt, but there is no equivalent to the Michelson-Morley experiment that can put it in serious doubt. Indeed, that was my intent with the prediction of the second peak, but cosmologists opted to abandon BBN (as it was then known) rather than accept the result. There always seems to be a way out, and that is always preferable to reexamining one’s belied system.
LikeLike
how soon will JWST provide data and specifically data on galaxies red shift ?
LikeLike
this may be of interest on the JWST Primordial black holes at high redshift
The James Webb telescope’s mission will be to find the first galaxies that formed in the early universe and see stars forming planetary systems.
Best of all, the existence of primordial black holes can be proven—or disproven—in the near future, courtesy of the James Webb Space Telescope and ESA’s Laser Interferometer Space Antenna (LISA) mission announced for the 2030s.
If dark matter is comprised of primordial black holes, more stars and galaxies would have formed around them in the early universe—precisely the epoch that the James Webb telescope will be able to see.
https://phys.org/news/2022-01-black-holes-dark-matterare.html
https://arxiv.org/abs/2109.08701
could dark matter is comprised of primordial black holes and MOND both be correct ?
LikeLike
Personally I don’t think the solution to the missing mass problem will involve primordial black holes or any form of dark matter. Requiring both dark matter and MOND seems to introduce as many problems as it solves. You don’t get to cherry pick the best bits of both. I suspect the problems with MOND will be overcome with an an overarching solution that reduces to MOND at galaxy scales while also explaining the additional mass discrepancy at cluster scales.
LikeLike
It’ll take some months to set up and calibrate. After that, deep imaging will commence almost immediately, though I don’t try to keep up with the complicated details of scheduling. The folks at STScI do a great job of this, and I find it best not to act like an overeager kid poking at the Xmas presents: the data will come soon enough. The imaging is relatively straightforward; what is harder are spectral redshifts. The faintest galaxies are always the hardest, and often require extensive follow up from ground based telescopes.
LikeLiked by 1 person
Will it also be possible to determine the rotation curves of these distant galaxies (i.e. to check if they too fall on the BTFR?)
I ask this because Mike McCulloch of quantized inertia predicts that galaxies at high z rotate faster because his minimum acceleration depends on the radius of the universe while MoND should not be influenced by the radius (in its current formulations).
LikeLike
Determining rotation curves is harder than imaging or just getting a spectroscopic redshift. For most high-z galaxies, it will not be possible. It will for some, and has already been done in a few cases (e.g., https://ui.adsabs.harvard.edu/abs/2021Sci…371..713L/abstract). But we need to see more of the host galaxies (JWST will help) and measure further out in each (JWST might help but probably not so much). Certainly it will be interesting to see how and if the BTFR evolves with redshift. Aside from simply getting the data, the challenge there is to account for stellar evolution. Galaxies in the early universe will be made of younger stars with lower mass-to-light ratios. Getting the translation to stellar mass is always an issue, as is measuring gas mass (no help from JWST for that).
LikeLiked by 1 person
Thank you for the answer.
Another question if you hazard to predict this – Will JWST see elliptical galaxies?
In my understanding, in MoND, galaxy mergers happen in timescales larger than in LCDM, but since MoND creates structure at an (much) earlier time, we can see basically the same elliptical galaxies abundance in both LCDM and MoND.
But at high z, if my understanding is correct, MoND predicts large spiral galaxies, but expects fewer ellipticals.
LikeLike
As a note to my previous question – I know it is difficult to create structure formation in MoND, but is it possible to place some bounds on the expected abundance for spirals vs ellipticals at the distance observable by JWST.
LikeLike
Just a correction – the above comment should read that it is difficult to SIMULATE structure formation.
LikeLike
Good question, which depends on the formation mechanism for ellipticals. These can form through mergers, but it doesn’t have to happen that way. There is a stability threshold in MOND that precludes disk galaxies with accelerations >> a0. I can imagine that as gas dissipates to form galaxies, some settle gently enough to make spirals, while others trip over this limit and violently relax as envisioned by Lynden-Bell. So my knee-jerk prediction is that we’ll see both spirals and ellipticals at high z. While it is true that merger times are longer in MOND, I don’t expect we’ll have to wait for all the ellipticals to form via this channel.
LikeLike
Similar conclusion form a gravity based approach. Different analysis. Alexandre Deur, “Effect of gravitational field self-interaction on large structure formation” arXiv: 2018:04649 (July 9, 2021) (Accepted for publication in Phys. Lett. B) DOI: 10.1016/j.physletb.2021.136510
LikeLike
Gravitational self interaction is the most likely solution in my view, though not necessarily Deur’s approach. My prediction is that JWST will show a link between missing mass and net gravitational acceleration. In addition the ratio of DM to Baryonic will be lower in the early universe due to its homogeneity.
LikeLike
re Big galaxies at high redshift! Big galaxies at high redshift!
If they find this, could the estimated age of the universe be wrong, and it is older than predicted, while preserving dark matter?
LikeLike
Good question. Yes, increasing the age of the universe would help. More technically, one might revisit the age-redshift relation, which depends on cosmological parameters. Those are pretty tied down, so not much wiggle room. However, we could always add new free parameters. The most obvious quasi-conventional way to do that would be through the dark energy equation of state. If that becomes necessary, you’ll see people proclaiming discoveries to this effect, that dark energy isn’t as simple as Einstein envisioned, but has some weird fluid time dependence. People have already considered such models; once you allow this freedom, you can fit pretty much any monotonic set of age-redshift data t(z). Ironically, many of these are modified gravity theories that only deal with the dark energy problem while taking a pass on the dark matter problem; I’d expect people to latch on to that before admitting that MOND predicted any of it. That’s what the community has done so far, apparently being happy to attribute to dark matter plus feedback plus anything else that’s necessary to “explain” after the fact what MOND predicted a priori.
LikeLike
thanks, yes that was the first thing in my mind.
LikeLike
Hi Stacy,
Thank you for this post on “What JWST will see”.
I am not going to speculate on what the James Webb Space Telescope (JWST) may or may not observe. I am pleased that the mission is progressing well and I’m more than happy to wait the 6 months or so for the first results to come in. Overall I am extremely satisfied that the observers have triumphed over the theorists and, in spite of all the predictions, seem to be following the mantra of “let’s simply go out and see what’s there”. Of course, they have prior observations from Hubble and are rightly confident that they will see something new. This is a much better position to be in than the particle physicists, who are clamouring for a bigger accelerator despite there being no prior data to indicate that anything new will be observed.
Where I do speculate is in the area of rocketry. Within a few years I expect follow up missions to JWST to be possible; for repairs, upgrades, and more coolant.
LikeLike
Look here, if a scientist is not supposed to spawn predictions, how will theoretic science ever make progress? Science without predictions says nothing, it isn’t even science. I think it’s great that Stacy is willing to stick out his neck as he does so that the truth will become clear. If he’s wrong, you have a reason to judge. But why judge before you even know whether he’s right?
If you’re really so set on the practical “shut up, just measure” approach, you shouldn’t even consider the prediction that if you’ve measured a result once then you will in the same situarion measure the same result the 2nd time.
LikeLike
could you comment on this
No need for dark matter: resolved kinematics of the ultra-diffuse galaxy AGC 114905
arXiv:2112.00017 [astro-ph.GA]
We present new HI interferometric observations of the gas-rich ultra-diffuse galaxy AGC 114905,…Only a halo with an extremely (and arguably unfeasible) low concentration reaches agreement with the data. We also find that the rotation curve of AGC 114905 deviates strongly from the predictions of Modified Newtonian dynamics. The inclination of the galaxy, which is measured independently from our modelling, remains the largest uncertainty in our analysis, but the associated errors are not large enough to reconcile the galaxy with the expectations of cold dark matter or Modified Newtonian dynamics.
LikeLike
I’ve seen some discussions about that too. It’s similar to talk about DF-2 and DF-4 imo. From what I understand about this paper in particular the problem is that the assumptions going into the analysis are rather questionable. The paper assumes the galaxy is circular like a squashed football so they can fit an inclination to it. There’s no reason it should be, especially not for such a tiny galaxy. Just look at figure 1b. That doesn’t look like a smooth matter distribution to me. There’s actual holes it it and everything. So I don’t know why the authors assume it is reasonable to assume this galaxy has a regular shape they can fit for. Furthermore mass scales in MOND as 1/sin^4(i), so a fourth power of inclination. Even a small change in inclination will have large changes in the inferred mass. Given their shaky assumptions I don’t think it is fair to take their stated error margins as fact considering that a small change of inclination would change the outcome of their analysis.
LikeLike
thanks. and for DF-2 and DF-4 it could be explained in terms of external field effect, if MOND is correct?
LikeLike
I glad to see that JWST has completed the last of its unfolding safely with the primary mirror in its final position. Now it is a matter of getting all the hexagonal mirrors in the right position relative to each other. They also think that the fuel will last for 20 years, not 10 (Note to David Carnochan: it’s fuel for keeping the telescope in a halo orbit around L2; the telescope and instruments are passively cooled by radiation to the cold sky in the direction away from the Sun)
https://www.bbc.co.uk/news/science-environment-59914936
It would be rather nice if one of their early images was of the Hubble ultra deep field, as the different wavelengths would give us useful information about something we already know about.
LikeLiked by 1 person
is there any kind of data JWST could in principal see in the near-infrared that would provide very strong support for MOND that can’t be easily explained for example by changing the age of the universe?
OTOH
there are papers which suggest JWST could identify dark matter as entirely as primordial black holes in a certain mass range
arXiv:2109.08701
Detection of the deep IR source count distribution by the JWST could reveal the existence of this population of high-redshift star-forming and accreting PBH DM.
LikeLike